Gazing deeply
PUBLISHED: 01 Jun 2012 00:31:27 | UPDATED: 06 Jun 2012 00:46:47PUBLISHED: 01 Jun 2012 PRINT EDITION: 01 Jun 2012Bryan Gaensler
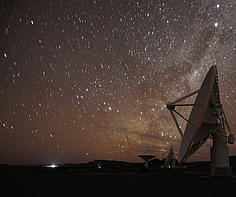
Radio telescopes, Carnarvon, South Africa - the site for the propsed square kilometre array. Mke Hutchings/Reuters
For thousands of years, the sole focus of astronomy was gazing up at the stars. In 1609, Galileo pointed a telescope at the sky and modern astronomy was born.
But since the 1930s, light has not been our only way of studying the sky. Stars and galaxies also emit radio waves, a realisation that has provided us with a powerful way of studying otherwise invisible aspects of the cosmos.
In the decades after World War II, Australia led the way in building a series of ever larger dishes designed to detect faint radio waves from the sky. Scientists soon realised that they could build arrays of many such dishes, and then could link them together electronically to further improve their resolving power and sensitivity.
The discoveries with these new telescopes streamed in: radio astronomers won Nobel Prizes in 1974, 1978 and 1993. But as the details of the radio sky began to be fleshed out and the pace of discovery slowed, the community began to think ahead to the next big thing.
In October 1990, just as I was finishing up at high school, British astronomer Peter Wilkinson gave a provocative talk at a conference in New Mexico. Wilkinson’s presentation, modestly titled The Hydrogen Array, began with this statement: “The time is ripe for planning an array with a collecting area of one square kilometre.”
At around the same time, other astronomers in the Netherlands, India and Canada independently proposed a similar idea. The consensus emerged that the remaining big questions in astrophysics and cosmology could not be answered with a marginally bigger dish or a slightly larger array, but demanded a whole new way of doing astronomy: a vast facility, spanning an entire continent, with a data stream so massive that it would require the world’s most powerful supercomputer to handle it.
The power of what is now called the Square Kilometre Array comes from the dishes being widely separated but linked electronically to simulate the resolving power of a giant telescope. By spreading elements of the SKA across thousands of kilometres, we create a spectacular continental-sized lens, capable of seeing far finer detail even than the Hubble Space Telescope.
The idea that began as the Hydrogen Array became the “1kT”, then the “SKAI”, and finally the Square Kilometre Array (SKA): an international collaboration from the outset, and a grassroots idea that has evolved into a multibillion-dollar mega-science project.
Fast forward 22 years from Wilkinson’s talk, past dozens of international meetings, hundreds of teleconferences, and tens of thousands of pages of memos, white papers and other documentation. We now find ourselves embarking on the final phase of this epic project – two sites have been selected for different components of the project (in southern Africa and in Australia/ New Zealand), and design and construction can begin.
So what’s all the fuss? What is it about the SKA concept that has rallied so many astronomers, engineers and now politicians to its cause? What the SKA promises are definitive answers to frustrating, basic questions about the world around us. We take it for granted that we know so much, but there are basic things for which our understanding is embarrassingly limited.
Take gravity, for instance. We know what gravity does – it makes apples fall, and it forces the Earth to orbit the sun. We can make exquisite predictions based on our understanding of its strength and its consequences – for example, we can say with absolutely certainty, down to the nearest second, when every solar eclipse for hundreds of years hence will occur.
But just what is gravity? Well, we’re not completely sure. Our current best understanding comes from Albert Einstein’s Theory of General Relativity, which says that gravity is a curvature in space-time produced by massive bodies. This might not seem obvious or make a whole lot of sense, but General Relativity makes some pretty weird but specific predictions, and has passed every test we’ve been able to throw at it with flying colours.
Many might think that the matter is settled: Einstein was right (as he almost always was!) and General Relativity correctly describes gravity. But by the standards of the cosmos, the tests we’ve applied to General Relativity have been downright pathetic, akin to testing a claim of a fireproof safe by holding a match against it. To understand gravity, we need to push it to its breaking point, and the SKA is the way to do this.
Somewhere amongst the hundreds of billions of stars in our Milky Way galaxy, sheer weight of numbers guarantees that the most exotic concoctions imaginable all exist by the truckload. The Holy Grail amongst these is the ultimate odd couple: a neutron star orbiting a black hole. Neutron stars emit faint radio waves, and black holes have extreme gravity, so together such a pairing provides the perfect test: General Relativity tells us there should be all sorts of strange deviations of the orbiting star from a simple circular path due to the extreme environment of a black hole, and tells us what these deviations should be to multiple decimal places. If we have a radio telescope that can pick up the neutron star’s signals as it orbits, we can see whether the predictions of General Relativity are correct. A neutron star around a black hole is to gravity what an armada of flame-throwers is to our fireproof safe.
Only problem? We don’t yet know of any neutron stars orbiting black holes, and even if we did, we wouldn’t be able to make the precise measurements needed to test our theories of gravity. Enter the SKA, a facility that will have the massive field-of-view needed to search the entire galaxy looking for such a rare pairing of objects, and that will also provide the extraordinary sensitivity required to then exquisitely track the path of the neutron star in its orbit. With the SKA, we will either finally confirm that our most basic understanding of the most fundamental physical force is correct, or we will be forced to put General Relativity on the scrapheap and will need to rebuild the foundations of physics from scratch.
The above is a very focused experiment, but it’s just one facet of what the SKA offers. How did the first stars and galaxies form, 13 billion years ago? Where does magnetism come from? What is Dark Energy, the mysterious repulsive force that is causing the entire universe to accelerate in its expansion? And arguably the biggest question of all – are there other planets out there like Earth, and do they harbour intelligent life, like us? The unique resolving power, enormous collecting area and spectacular wide-angle view that the SKA will provide will let us answer all these questions, plus will provide many more answers to questions we haven’t even thought of yet.
Soon the SKA’s arrays of dishes and antennas will begin taking shape in remote parts of Africa and Australasia. By 2020, data will begin to flow, and some stunning new images of the radio sky will emerge. I hope Peter Wilkinson and his visionary colleagues are among those who get the first look at this brave new frontier.
Bryan Gaensler is an Australian laureate fellow at the University of Sydney, and is director of the Centre of Excellence for All-sky Astrophysics. He was formerly the international project scientist for the Square Kilometre Array. Twitter: @SciBry
The Australian Financial Review